What is the difference between a mitochondria ATP synthase F1 rotor spinning at max efficiency and one that is slowed by high matrix viscosity? The difference is ~40000 rotations per min vs ~8 rpm. At 3 ATP molecules hydrolyzed per rotation, the difference is 120000 or 24 ATPs.
Doris Loh
@DorissLoh
That is how melatonin can enhance ATP production: by lowering matrix viscosity to elevate not only ATP synthase F1 rotor spin speed, but also expression and activity of complex IV (COX). Want to find out more? Read this groundbreaking paper on melatonin.
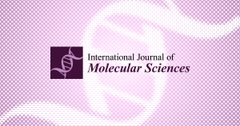
mdpi.com
Light, Water, and Melatonin: The Synergistic Regulation of Phase Separation in Dementia
The swift rise in acceptance of molecular principles defining phase separation by a broad array of scientific disciplines is shadowed by increasing discoveries linking phase separation to pathologi…
4:21 PM · Apr 2, 2023
·
143
Views
====
Favorites · ·
My latest peer-reviewed paper talked about the importance of lowering viscosity in mitochondria matrix [1]. Why is that important for health?
Lower viscosity can allow the ATPase rotor to spin faster to produce more ATP molecules to support biological functions.
What you may not know is how fast the ATPase F1 rotor actually spins. Most people say the ATP synthase spins at about 9000 rotations per minute, which is about 150 rotations per second. That is super fast, right? Wrong.
According to the results reported in a peer-reviewed study published in 2006 [2] where the authors attached a gold nanobead to track the rotation of the F1 rotor of the ATP synthase in the bacteria Escherichia coli, the F1 rotor can spin at minimum average speed of 380 rotations per SECOND. The results totally shocked the authors who expected a rotation of merely 30 rps.
At maximum, the authors recorded a speed of 671 rps, which means the ATP synthase F1 motor rotates at 40,260 times per minute. Can you imagine what changes in viscosity can do to the speed of rotation in mitochondria ATP synthase?
Variations of up to 5000-fold can be induced by changes in viscous drag, causing the rotor to spin at slower speeds. So at maximum, your mitochondria ATP rotors can be spinning at 40,000 rotations per minute, but at minimum, it could be rolling around at 8 rotations per minute reflecting a 5000-fold reduction [2].
Now do you understand why higher matrix viscosities are associated with all types of illnesses including cancer and neurodegeneration, and why melatonin, being able to reduce viscosity in mitochondrial matrix, can increase ATP production to reverse basically any health challenge imaginable?
If you haven’t read my ground-breaking paper or shared it with your health gurus and/or doctors and healthcare practitioners, perhaps it is time you do so. https://www.mdpi.com/1422-0067/24/6/5835
Have you had your MEL and AA today?*
- Disclaimer: These statements have not been evaluated by the Food and Drug Administration. Ascorbic acid, melatonin and any other product mentioned is not intended to diagnose, treat, cure or prevent any disease.
References:
[1] Loh, D.; Reiter, R.J. Light, Water, and Melatonin: The Synergistic Regulation of Phase Separation in Dementia. Int. J. Mol. Sci. 2023, 24, 5835. https://doi.org/10.3390/ijms24065835
[2] Nakanishi-Matsui, M.; Kashiwagi, S.; Hosokawa, H.; Cipriano, D. J.; Dunn, S. D.; Wada, Y.; Futai, M. Stochastic High-Speed Rotation of Escherichia Coli ATP Synthase F1 Sector: The Epsilon Subunit-Sensitive Rotation. J. Biol. Chem. 2006, 281, 4126–4131.